MCP6271R Microchip Technology Inc., MCP6271R Datasheet - Page 10
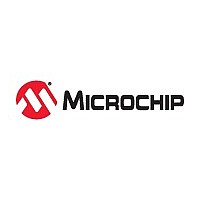
MCP6271R
Manufacturer Part Number
MCP6271R
Description
170 ?a, 2 Mhz Rail-to-rail Op Amp
Manufacturer
Microchip Technology Inc.
Datasheet
1.MCP6271R.pdf
(40 pages)
Available stocks
Company
Part Number
Manufacturer
Quantity
Price
Company:
Part Number:
MCP6271RT-E/OT
Manufacturer:
MCHP
Quantity:
6 004
Part Number:
MCP6271RT-E/OT
Manufacturer:
MICROCHIP/微芯
Quantity:
20 000
Charging High Capacity Lithium Batteries
By George Paparrizos, Microchip Technology Inc.
New portable electronic designs are continuously improving
system performance. Designers are adding new features, and the
power requirements of these systems are increasing along with
their performance. This trend has resulted in the development
of Lithium Ion (Li-Ion) battery packs with significantly higher
capacities that can provide adequate system run times. Li-
Ion batteries have emerged to be the power source of choice
for most portable consumer applications. They provide many
advantages over other battery types, such as higher energy
density and cell voltages. However, as the available battery
capacity increases to address today’s growing power demands,
consumer requirements for shorter battery-charge cycles
and smaller physical size are also increasing. Meeting those
requirements becomes a major design challenge.
The preferred charging rate for a Li-Ion or Li-Polymer battery
pack is 1C, which means that a 1000 mAh battery pack has
a preferred fast-charge current of 1A. Charging at this rate
provides the shortest charge-cycle times without degradation to
the battery-pack performance or its life. To achieve the preferred
charging rate while utilizing battery cells with increasingly higher
capacity, a higher charge-current level is inevitable. This leads
to major design challenges, the most important of which is the
heat generated in the charge-management controller IC as well
as on the system board. To address these issues, a more careful
thermal design is necessary. As shown below, integrating the
pass transistor, which dissipates most of the heat, on the charge
management IC, becomes, in many cases, a limiting factor for
the design performance and operating range.
The power dissipation of a linear-battery charger, the most
popular type for 1- and 2-cell Lithium-based applications, can be
calculated as follows:
With:
P
V
V
I
The above formula indicates that a worst-case scenario exists
when the input-voltage to battery-voltage differential and the
charge current are high. We calculate the power dissipation for
a typical scenario, in which the car or wall adapter provides a 5V
±10 percent supply voltage, the battery voltage is 3.0V and the
fast-charge current is 1A. (i.e., 1C charging rate for a 1000 mAh
battery) In this case, the power dissipation is calculated to:
Table 1 shows the maximum operating ambient temperature
assuming a 2.5W power-dissipation scenario, and a maximum
junction temperature of 125°C, for some of the most popular
package options in the industry.
8
CHARGE
Analog and Interface Guide – Volume 2
D
DD
BATTERY
: power dissipation
: input (adapter) voltage
Charging Lithium Batteries
P
P
D
D
: fast charge current
= (V
= (5.5V – 3.0V) x 1A = 2.5W
: battery voltage
DD
- V
BATTERY
) x I
CHARGE
It is apparent from these results that the maximum allowable
ambient temperature for most of the package types puts
significant limitations on the thermal design. Furthermore,
achieving some of these operating conditions is not feasible in
a real-world situation. The assumptions for conducting these
calculations do not take into account worst-case conditions.
For example, the battery might have just been preconditioned
(i.e. deeply depleted cells) in which case the battery voltage
during the beginning of current regulation could be as low as
2.7V. Additionally, after-market adapters that provide a less tight
regulation might be utilized, such that the battery charger would
accept input voltages significantly higher than 5V. Lastly, as new
applications require more power, battery packs with capacities
greater than 1000 mAh are becoming increasingly popular.
Some battery chargers, like Microchip Technology’s MCP7384X,
provide the ability to use an external P-channel MOSFET. Discrete
MOSFETs can run hotter without reliability concerns. They are
offered in a variety of heat-dissipating packages that enable
higher allowable power dissipation as well as higher maximum
operating ambient temperature. The flexibility of selecting a
MOSFET that meets the application’s power dissipation and
electrical requirements allows for higher system performance and
cost optimization. Figure 1 demonstrates a typical circuit for this
battery-charger family.
The MCP7384X also offers a ±0.5 percent voltage regulation
accuracy (max). Since both over- and under-charging the battery
reduces its performance and life, it is essential for the charge
management controller IC to provide a high-precision voltage
to the battery. Exceeding the termination voltage allows for a
short-term gain in available battery energy. However, continuous
overcharging can result in a significant reduction in cycle life or
safety issues. On the other hand, undercharging reduces the
battery cell’s available capacity, thereby shortening usable battery
time; thus, providing a precise regulation voltage maximizes cell
capacity utilization and ensures safe operation. Figures 2 and 3
illustrate that a small decrease in voltage accuracy results in a
large decrease in battery capacity.
Table 1: Max. Operating Ambient Temperature
(2.5W Power-Dissipation, Max. Junction Temperature 125°C)
MSOP-8
SOIC-8
MSOP-10
SOT-23-6
3x3 DFN-8
Power SOIC-8
4x4 QFN-16
5x6 DFN-8
Package
Type
Thermal Resistance
(°C/W)*
206
163
113
96
52
43
38
36
Temperature (°C)
Max. Ambient
-391
-283
-158
-114
18
30
35
-5