OPA642N BURR-BROWN [Burr-Brown Corporation], OPA642N Datasheet - Page 12
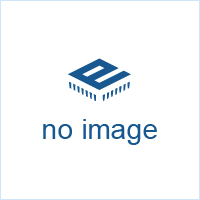
OPA642N
Manufacturer Part Number
OPA642N
Description
Wideband, Low Distortion, Low Gain OPERATIONAL AMPLIFIER
Manufacturer
BURR-BROWN [Burr-Brown Corporation]
Datasheet
1.OPA642N.pdf
(15 pages)
Available stocks
Company
Part Number
Manufacturer
Quantity
Price
Part Number:
OPA642N/250
Manufacturer:
TI/德州仪器
Quantity:
20 000
Part Number:
OPA642NB/250
Manufacturer:
TI/德州仪器
Quantity:
20 000
configuration this is sum of R
configuration this is just R
voltage swing increases harmonic distortion directly. A 6dB
increase in output swing will generally increase the second
harmonic 12dB and the third harmonic 18dB. Increasing the
signal gain will also increase the second harmonic distortion.
Again a 6dB increase in gain will increase the second and third
harmonic by 6dB even with a constant output power and
frequency. And finally, the distortion increases as the funda-
mental frequency increases due to the rolloff in the loop gain
with frequency. Conversely, the distortion will improve going
to lower frequencies down to the dominant open-loop pole at
approximately 3kHz. Starting from the –90dBc second har-
monic for 2Vp-p into 500 , G = +2 distortion at 1MHz (from
the Typical Performance Curves), the second harmonic distor-
tion at 20kHz should be approximately –90dB – 20log
(1MHz/20kHz) = –124dBc.
The OPA642 has an extremely low third order harmonic
distortion. This also gives an exceptionally good two-tone,
third-order intermodulation intercept as shown in the Typi-
cal Performance Curves. This intercept curve is defined at
the 50
to allow direct comparisons to RF MMIC devices. This
network attenuates the voltage swing from the output pin to
the load by 6dB. If the OPA642 drives directly into the input
of a high impedance device, such as an ADC, this 6dB
attenuation is not taken. Under these conditions, the inter-
cept will increase by a minimum 6dBm. The intercept is
used to predict the intermodulation spurious for two closely
spaced frequencies. If the two test frequencies, f1 and f2, are
specified in terms of average and delta frequency, f
+ f2)/2 and f = |f
spurious tones will appear at f
between two equal test tone power levels and these
intermodulation spurious power levels is given by 2 • (IM3
– P
Performance Curve and P
50 load for one of the two closely spaced test frequencies.
For instance, at 10MHz the OPA642 at a gain of +2 has an
intercept of 46dBm at a matched 50
envelope of the two frequencies needs to be 2Vp-p, this
requires each tone to be 4dBm. The third-order
intermodulation spurious tones will then be 2 • (46 – 4) =
84dBc below the test tone power level (–80dBm). If this
same 2Vp-p two-tone envelope were delivered directly into
the input of an ADC without the matching loss or loading of
the 50
52dBm. With the same signal and gain conditions but now
driving directly into a light load, the spurious tones will then
be at least 2 • (52 – 4) = 96dBc below the 1Vp-p test tone
signal levels.
NOISE PERFORMANCE
The OPA642 complements its ultra-low harmonic distortion
with low input noise terms. Both the input referred voltage
noise, and the two input referred current noise terms com-
bine to give a low output noise under a wide variety of
operating conditions. Figure 6 shows the op amp noise
analysis model with all the noise terms included. In this
O
) where IM3 is the intercept taken from the Typical
load when driven through a 50 matching resistor
network, the intercept would increase to at least
®
OPA642
2
– f
2
|
O
is the power level in dBm at the
F
F
(Figure 1). Increasing output
the two, third-order, close-in
O
+ R
G
(3 • f). The difference
, while in the inverting
load. If the full
O
= (f1
12
model, all the noise terms are taken to be noise voltage or
current density terms in either nV/ Hz or pA/ Hz.
The total output spot noise voltage can be computed as the
square root of the squared contributing terms to the output
noise voltage. This computation is adding all the contribut-
ing noise powers at the output by superposition, then taking
the square root to get back to a spot noise voltage. Equation
1 shows the general form for this output noise voltage using
the terms shown in Figure 6.
FIGURE 6. Op Amp Noise Analysis Model.
Dividing this expression by the noise gain (G
will give the equivalent input referred spot noise voltage at
the non-inverting input as shown in Equation 2.
Evaluating these two equations for the OPA642 circuit
shown in Figure 1 will give a total output spot noise voltage
of 6.7nV/ Hz and an equivalent input spot noise voltage of
3.35nV/ Hz.
Narrowband communications systems are more commonly
concerned with the noise figure for the amplifier. The total
input referred voltage noise expression (Eq. 2), may be used
to calculate the noise figure. Equation 3 shows this noise
figure expression using the E
configuration where the input terminating resistor R
set to match the source impedance (as shown in Figure 1).
Evaluating Equation 3 for the circuit of Figure 1 gives a
Noise Figure = 17.6dB. Input transformer coupling can be
used to reduce this noise figure. A broadband pulse trans-
former can provide both a noiseless voltage gain and a more
optimum source impedance to minimize the noise figure.
Figure 7 shows an example built from the circuit of Figure
1, in which the transformer turns ratio has been set to the
E
E
E
O
RS
R
N
S
E
4kTR
4kT
R
E
NI
G
NI
2
S
2
I
I
BN
BN
I
NF 10 log 2
BN
E
R
NI
S
R
2
S
2
4kTR
R
G
4kTR
N
OPA642
of Eq. 2 for the non-inverting
S
G
I
S
N
BI
kTRs
E
2
R
F
N
2
4kT = 1.6E –20J
I
I
BI
G
BI
at 290°K
4kTR
R
R
N
F
F
N
2
F
2
= 1 + R
4kTR
T
4kTR
has been
G
F
N
F
Eq. 1
Eq. 2
Eq. 3
/R
G
E
F
N
O
G
)