LTC1775 LINER [Linear Technology], LTC1775 Datasheet - Page 10
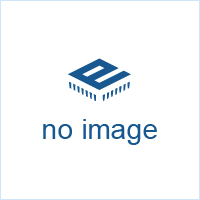
LTC1775
Manufacturer Part Number
LTC1775
Description
High Power No RSENSE TM Current Mode Synchronous Step-Down Switching Regulator
Manufacturer
LINER [Linear Technology]
Datasheet
1.LTC1775.pdf
(24 pages)
Available stocks
Company
Part Number
Manufacturer
Quantity
Price
Company:
Part Number:
LTC1775CGN
Manufacturer:
LT
Quantity:
10 000
Part Number:
LTC1775CGN
Manufacturer:
LINEAR/凌特
Quantity:
20 000
Part Number:
LTC1775CGN#PBF
Manufacturer:
LINEAR/凌特
Quantity:
20 000
Part Number:
LTC1775CS
Manufacturer:
LINEAR/凌特
Quantity:
20 000
Part Number:
LTC1775CS#PBF
Manufacturer:
LINEAR/凌特
Quantity:
20 000
Part Number:
LTC1775CS#TRPBF
Manufacturer:
LINEAR/凌特
Quantity:
20 000
Company:
Part Number:
LTC1775IGN
Manufacturer:
LT
Quantity:
10 000
APPLICATIO S I FOR ATIO
LTC1775
paid to the resulting thermal issues. Under DC conditions,
the maximum power that can be dissipated by a MOSFET
switch limits the current through it:
For example, the SUD50N03-10 with T
T
can operate with a maximum DC current of 10A. In a
switching application, the actual power dissipation is
increased by the transition losses and is reduced by the
switch duty cycle. When the LTC1775 is operating in
continuous mode, the duty cycles for the MOSFETs are:
The MOSFET power dissipations at maximum output
current are:
Both MOSFETs have I
includes an additional term for transition losses, which are
largest at high input voltages. The constant k = 1.7 can be
used to estimate the amount of transition loss. The bottom
MOSFET losses are greatest at high input voltage or during
a short circuit when the duty cycle is nearly 100%. The
temperature rise of the MOSFETs depends on the effective
thermal resistance
tion. Check the temperature of the MOSFET when testing
applications and use appropriate heat sinking such as
board power planes to spread the heat.
10
A
I
=70 ,
Bottom Duty Cycle
P
P
Top Duty Cycle
DS MAX
BOT
TOP
(
JA
)
V
V
= 30 C/W, R
( )(
V
OUT
IN
k V
IN
R
–
V
DS ON
IN
IN
V
P
U
(
(
2
OUT
I
JA
O MAX
)(
V
V
(
I
)
OUT
of the heat sink used in the applica-
2
O MAX
IN
R losses and the P
(
V
(
U
DS(ON)
I
IN
O MAX
)
2
(
V
–
)(
)
IN
)(
V
JA DS ON
C
T TOP
OUT
)
= 0.019 ,
(
2
RSS
R
T
)(
J MAX
W
(
)( )
(
)
T BOT
)(
(
f
R
)
)
J(MAX)
DS ON
–
)
TJ MAX
T
(
)(
TOP
TJ(MAX)
A
(
R
U
)
DS ON
= 175 C,
)
equation
(
)
= 1.8
)
)
Operating Frequency and Synchronization
The choice of operating frequency and inductor value is a
trade-off between efficiency and component size. Low
frequency operation improves efficiency by reducing
MOSFET switching losses, both gate charge loss and
transition loss. However, lower frequency operation
requires more inductance for a given amount of ripple
current.
The internal oscillator runs at a nominal 150kHz frequency
when the SYNC pin is left open or connected to ground.
Pulling the SYNC pin above 1.2V will increase the fre-
quency by 50%. The oscillator will injection lock to a clock
signal applied to the SYNC pin with a frequency between
165kHz and 200kHz. The clock high level must exceed
1.2V for at least 1 s and no longer than 4 s as shown in
Figure 4. The top MOSFET turn-on will synchronize with
the rising edge of the clock.
Inductor Value Selection
Given the desired input and output voltages, the inductor
value and operating frequency directly determine the
ripple current:
Lower ripple current reduces losses in the inductor, ESR
losses in the output capacitors and output voltage ripple.
Thus, highest efficiency operation is obtained at low
frequency with small ripple current. To achieve this, how-
ever, requires a large inductor.
I
L
0
( )( )
V
f L
1 s < t
OUT
Figure 4. SYNC Clock Waveform
ON
7V
1.2V
< 4 s
– 1
5 s < t < 6 s
V
V
OUT
IN
1775 F04